Volume 5 Issue 3 2024
Click Here to Download Full IssueEDITORIAL
2024, 5(3): 203–204. doi:https://doi.org/10.12336/biomatertransl.2024.03.001
REVIEW
2024, 5(3): 205–235. doi:https://doi.org/10.12336/biomatertransl.2024.03.002
Bone defects are a prevalent category of skeletal tissue disorders in clinical practice, with a range of pathogenic factors and frequently suboptimal clinical treatment effects. In bone regeneration of bone defects, the bone regeneration microenvironment—composed of physiological, chemical, and physical components—is the core element that dynamically coordinates to promote bone regeneration. In recent years, medical biomaterials with bioactivity and functional tunability have been widely researched upon and applied in the fields of tissue replacement/regeneration, and remodelling of organ structure and function. The biomaterial treatment system based on the comprehensive regulation strategy of bone regeneration microenvironment is expected to solve the clinical problem of bone defect. Hydrogel microspheres (HMS) possess a highly specific surface area and porosity, an easily adjustable physical structure, and high encapsulation efficiency for drugs and stem cells. They can serve as highly efficient carriers for bioactive factors, gene agents, and stem cells, showing potential advantages in the comprehensive regulation of bone regeneration microenvironment to enhance bone regeneration. This review aims to clarify the components of the bone regeneration microenvironment, the application of HMS in bone regeneration, and the associated mechanisms. It also discusses various preparation materials and methods of HMS and their applications in bone tissue engineering. Furthermore, it elaborates on the relevant mechanisms by which HMS regulates the physiological, chemical, and physical microenvironment in bone regeneration to achieve bone regeneration. Finally, we discuss the future prospects of the HMS system application for comprehensive regulation of bone regeneration microenvironment, to provide novel perspectives for the research and application of HMS in the bone tissue engineering field.
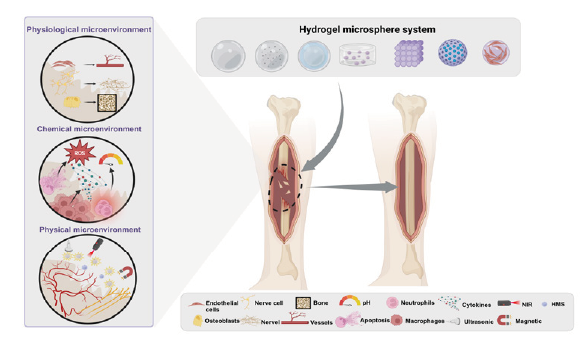
REVIEW
2024, 5(3): 236–256. doi:https://doi.org/10.12336/biomatertransl.2024.03.003
Bone, cartilage, and soft tissue regeneration is a complex process involving many cellular activities across various cell types. Autografts remain the “gold standard” for the regeneration of these tissues. However, the use of autografts is associated with many disadvantages, including donor scarcity, the requirement of multiple surgeries, and the risk of infection. The development of tissue engineering techniques opens new avenues for enhanced tissue regeneration. Nowadays, the expectations of tissue engineering scaffolds have gone beyond merely providing physical support for cell attachment. Ideal scaffolds should also provide biological cues to actively boost tissue regeneration. As a new type of injectable biomaterial, hydrogel microspheres have been increasingly recognised as promising therapeutic carriers for the local delivery of cells and drugs to enhance tissue regeneration. Compared to traditional tissue engineering scaffolds and bulk hydrogel, hydrogel microspheres possess distinct advantages, including less invasive delivery, larger surface area, higher transparency for visualisation, and greater flexibility for functionalisation. Herein, we review the materials characteristics of hydrogel microspheres and compare their fabrication approaches, including microfluidics, batch emulsion, electrohydrodynamic spraying, lithography, and mechanical fragmentation. Additionally, based on the different requirements for bone, cartilage, nerve, skin, and muscle tissue regeneration, we summarize the applications of hydrogel microspheres as cell and drug delivery carriers for the regeneration of these tissues. Overall, hydrogel microspheres are regarded as effective therapeutic delivery carriers to enhance tissue regeneration in regenerative medicine. However, significant effort is required before hydrogel microspheres become widely accepted as commercial products for clinical use.
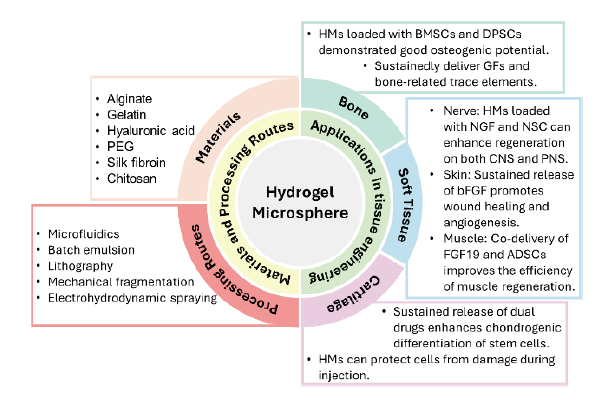
REVIEW
2024, 5(3): 257–273. doi:https://doi.org/10.12336/biomatertransl.2024.03.004
The treatment of bone defects remains a great clinical challenge. With the development of science and technology, bone tissue engineering technology has emerged, which can mimic the structure and function of natural bone tissues and create solutions for repairing or replacing human bone tissues based on biocompatible materials, cells and bioactive factors. Hydrogels are favoured by researchers due to their high water content, degradability and good biocompatibility. This paper describes the hydrogel sources, roles and applications. According to the different types of stimuli, hydrogels are classified into three categories: physical, chemical and biochemical responses, and the applications of different stimuli-responsive hydrogels in bone tissue engineering are summarised. Stimuli-responsive hydrogels can form a semi-solid with good adhesion based on different physiological environments, which can carry a variety of bone-enhancing bioactive factors, drugs and cells, and have a long retention time in the local area, which is conducive to a long period of controlled release; they can also form a scaffold for constructing tissue repair, which can jointly promote the repair of bone injury sites. However, it also has many defects, such as poor biocompatibility, immunogenicity and mechanical stability. Further studies are still needed in the future to facilitate its clinical translation.
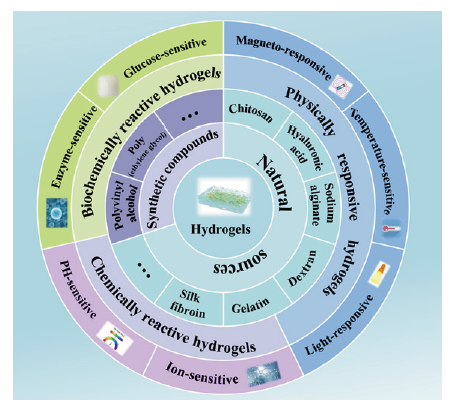
REVIEW
2024, 5(3): 274–299. doi:https://doi.org/10.12336/biomatertransl.2024.03.005
The treatment and repair of bone tissue damage and loss due to infection, tumours, and trauma are major challenges in clinical practice. Artificial bone scaffolds offer a safer, simpler, and more feasible alternative to bone transplantation, serving to fill bone defects and promote bone tissue regeneration. Ideally, these scaffolds should possess osteoconductive, osteoinductive, and osseointegrative properties. However, the current first-generation implants, represented by titanium alloys, have shown poor bone-implant integration performance and cannot meet the requirements for bone tissue repair. This has led to increased research on second and third generation artificial bone scaffolds, which focus on loading bioactive molecules and cells. Polymer microspheres, known for their high specific surface areas at the micro- and nanoscale, exhibit excellent cell and drug delivery behaviours. Additionally, with their unique rigid structure, microsphere scaffolds can be constructed using methods such as thermal sintering, injection, and microsphere encapsulation. These scaffolds not only ensure the excellent cell drug loading performance of microspheres but also exhibit spatial modulation behaviour, aiding in bone repair within a three-dimensional network structure. This article provides a summary and discussion of the use of polymer microsphere scaffolds for bone repair, focusing on the mechanisms of bone tissue repair and the current status of clinical bone grafts, aimed at advancing research in bone repair.
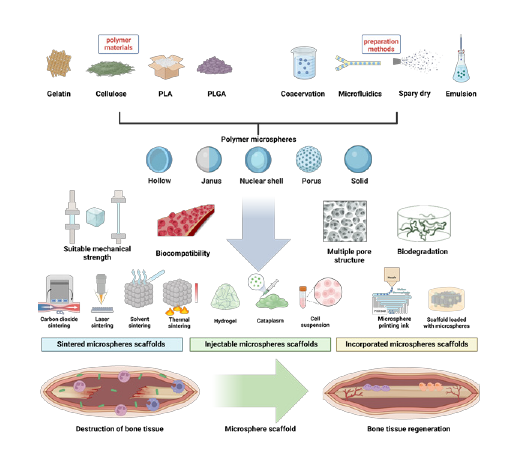
RESEARCH ARTICLE
2024, 5(3): 300–313. doi:https://doi.org/10.12336/biomatertransl.2024.03.006
Skull defects are common in the clinical practice of neurosurgery, and they are easily complicated by encephalitis, which seriously threatens the life and health safety of patients. The treatment of encephalitis is not only to save the patient but also to benefit the society. Based on the advantages of injectable hydrogels such as minimally invasive surgery, self-adaptation to irregularly shaped defects, and easy loading and delivery of nanomedicines, an injectable hydrogel that can be crosslinked in situ at the ambient temperature of the brain for the treatment of encephalitis caused by cranial defects is developed. The hydrogel is uniformly loaded with nanodrugs formed by cationic liposomes and small molecule drugs dexmedetomidine hydrochloride (DEX-HCl), which can directly act on the meninges to achieve sustained release delivery of anti-inflammatory nanodrug preparations and achieve the goal of long-term anti-inflammation at cranial defects. This is the first time that DEX-HCl has been applied within this therapeutic system, which is innovative. Furthermore, this study is expected to alleviate the long-term suffering of patients, improve the clinical medication strategies for anti-inflammatory treatment, promote the development of new materials for cranial defect repair, and expedite the translation of research outcomes into clinical practice.
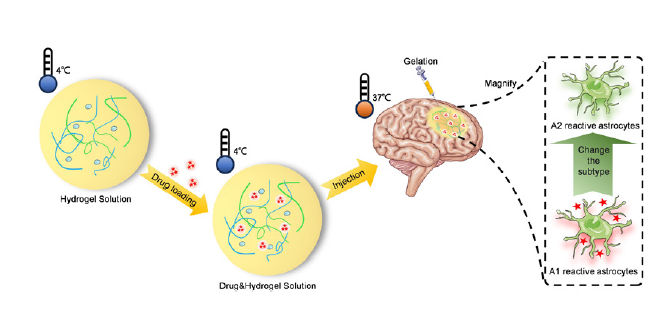
RESEARCH ARTICLE
2024, 5(3): 314–324. doi:https://doi.org/10.12336/biomatertransl.2024.03.007
Three-dimensional printed Ti-6Al-4V hemipelvic prosthesis has become a current popular method for pelvic defect reconstruction. This paper presents a novel biomimetic hemipelvic prosthesis design that utilises patient-specific anatomical data in conjunction with the Voronoi diagram algorithm. Unlike traditional design methods that rely on fixed, homogeneous unit cell, the Voronoi diagram enables to create imitation of trabecular structure (ITS). The proposed approach was conducted for six patients. The entire contour of the customised prosthesis matched well with the residual bone. The porosity and pore size of the ITS were evaluated. The distribution of the pore size ranged from 500 to 1400 μm. Porosity calculations indicated the average porosity was 63.13 ± 0.30%. Cubic ITS samples were fabricated for micrograph and mechanical analysis. Scanning electron microscopy images of the ITS samples exhibited rough surface morphology without obvious defects. The Young’s modulus and compressive strength were 1.68 ± 0.05 GPa and 174 ± 8 MPa, respectively. Post-operative X-rays confirmed proper matching of the customised prostheses with the bone defect. Tomosynthesis-Shimadzu metal artifact reduction technology images indicated close contact between the implant and host bone, alongside favourable bone density and absence of resorption or osteolysis around the implant. At the last follow-up, the average Musculoskeletal Tumour Society score was 23.2 (range, 21–26). By leveraging additive manufacturing and Voronoi diagram algorithm, customised implants tailored to individual patient anatomy can be fabricated, offering wide distribution of the pore size, reasonable mechanical properties, favourable osseointegration, and satisfactory function.
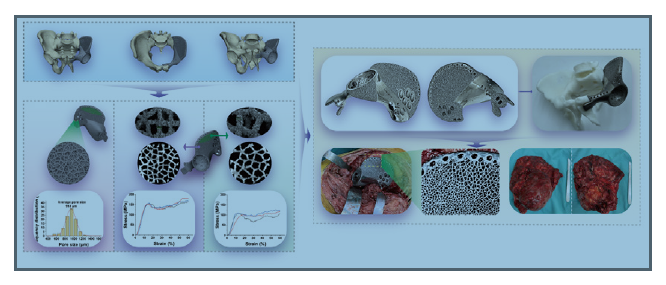
COMMENTARY
2024, 5(3): 325–327. doi:https://doi.org/10.12336/biomatertransl.2024.03.008
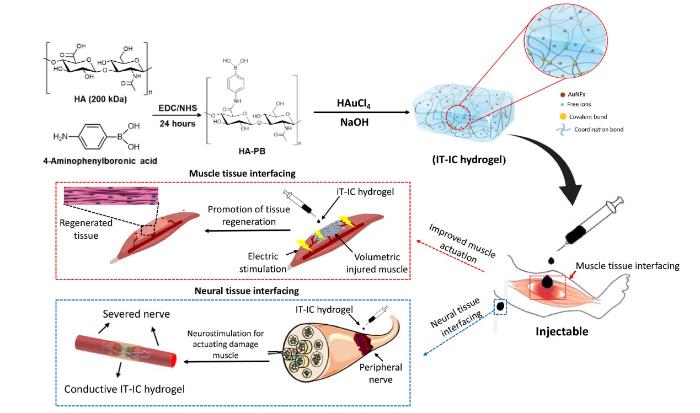
COMMENTARY
2024, 5(3): 328–330. doi:https://doi.org/10.12336/biomatertransl.2024.03.009
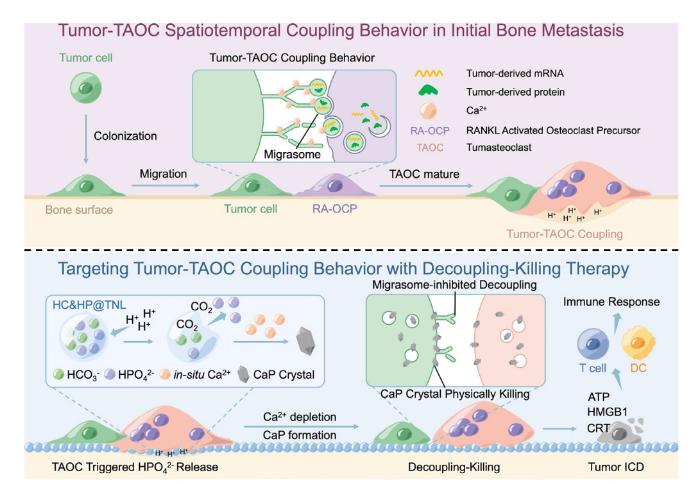
COMMENTARY
2024, 5(3): 331–333. doi:https://doi.org/10.12336/biomatertransl.2024.03.010
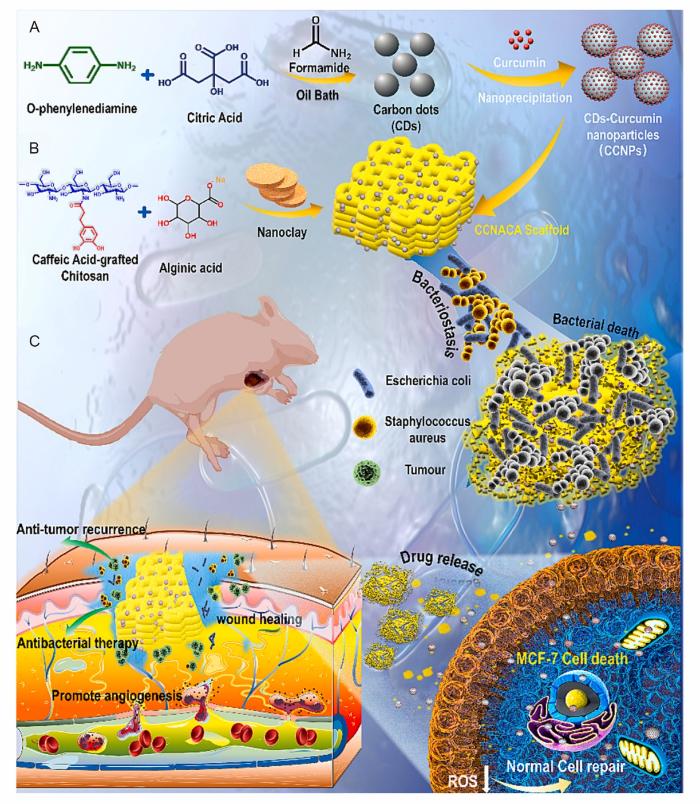